From Whence Comes the Quench
Beta decay plays an indispensable role in the universe. It drives the stellar explosions that synthesize elements—every piece of gold or platinum jewelry owes its beginnings to beta decay. It gives scientists a glimpse of physics beyond the Standard Model—the framework of particles and forces that gives a sense of order to the physical world. And for 50 years it has held onto a secret that puzzled nuclear physicists. With ever-advancing computing power at Oak Ridge National Laboratory, a team of researchers (including those with UT ties) has solved that mystery, with the results appearing in Nature Physics.
When a nucleus has too many protons or neutrons, a neutron will transform into a proton, or a proton will transform into a neutron. The process—beta decay—brings the nucleus closer to a region of stability. For half a century, nuclear physicists have been dogged by something of a problem of scale: why was it they could calculate the beta decay rate of a single neutron, but using that information for an atomic nucleus resulted in a too-fast decay?
Physics Professor Thomas Papenbrock, a co-author on the Nature Physics paper, explained that the adjustment for this discrepancy came in the form of “quenching”—reducing a fundamental coupling constant between decay rates for a free neutron and the decay rate scientists observed in an atomic nucleus. The puzzle was that there was no first-principles theoretical understanding for why quenching worked.
“Our work shows that the beta decay of a nucleus is more complicated,” Papenbrock said. “Even if we think of it as a decay of a neutron to a proton inside the nucleus, this decay is influenced by processes where two neutrons interact and transition into a proton and a neutron. Taking these effects into account, and using state-of-the art nuclear models and supercomputers, we were able to solve the puzzle of ‘quenched’ beta decays.”
Availing themselves of the Cray XK7 Titan Supercomputer at ORNL, the researchers simulated the decay of tin-100 into indium-100. Tin-100 is known as a “doubly-magic” nucleus: it has 50 protons and 50 neutrons in complete shells, making it strongly-bound. The relative simplicity of its structure makes tin-100 an ideal candidate for the sort of large-scale calculations where scientists need to understand the forces between the protons and neutrons in an atomic nucleus.
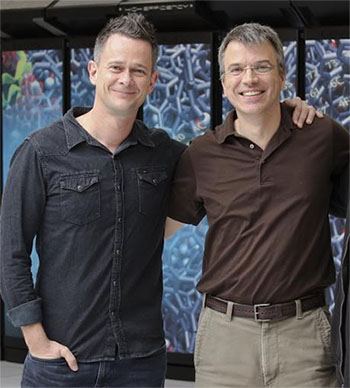
The results of this study are consistent with experimental data and could also inform predictions for the matrix element that governs neutrino-less double beta decay. This is a hypothetical process where two neutrons decay into protons at the same time, but without emitting neutrinos. Neutrino-less double beta decay, as the Nature Physics paper points out, has its own puzzle in terms of understanding the scale of mass in neutrinos, which up until 1998 were thought to have no mass at all.
The Nature Physics paper includes two authors with UT Physics ties: Papenbrock and Gaute Hagen (adjunct assistant professor). Please see the Oak Ridge National Laboratory press release for more detailed information about this research.
Pictured left: Gaute Hagen and Thomas Papenbrock (photo credit Oak Ridge National Laboratory).